ECO-DESIGN
Sustainable Industrial Engineering
In 2015, the 193 United Nations member states adopted the development program titled ‘Agenda 2030.’ This global roadmap aims to ‘Adapt industries to make them sustainable through rational resource use and increased reliance on clean and environmentally friendly industrial technologies and processes.’
A thorough understanding of materials, their production methods, transformation, and recycling is essential for developing the tools that will drive real changes in resource utilization and shaping technologies in the future.
The SUNI-Pft team supports your environmental transition projects, from eco-design to eco-manufacturing.
While awareness of environmental issues is well-established among individuals, local communities, and certain economic sectors (such as agriculture, construction, and energy), the same cannot be said for the industrial production sector. Indeed, the constraints of international competitiveness and evolving markets impose objectives related to quality, cost, lead time, and flexibility that constrain industries in their choices, leaving little room for other goals such as environmental or social performance.
However, the future of the production industry lies in better consideration of environmental and societal aspects, which presents a challenge to decision-makers of the next generation. Current energy and environmental crises clearly demonstrate the importance of rational resource utilization and waste management in production.
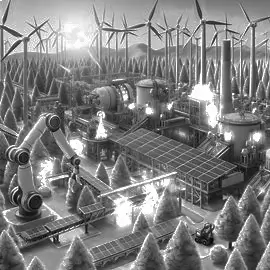
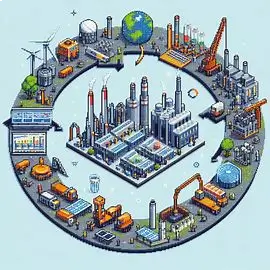
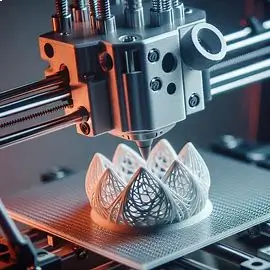
SUNI accompanies you in your environmental transition
Ecodesign
Traditionally, eco-design has been considered applicable to products (with historical examples related to packaging), but more recently, its scope has expanded to include services and systems. The term “product” is generally used in this broader sense, although the examples are typically material products.
Clearly, environmental constraints cannot be the sole focus of product study, at the expense of other essential factors such as customer expectations, technical feasibility, or cost control. In the eco-design process, the efficiency of a solution is measured not only by reducing environmental impacts but also by relating this reduction to the costs it entails; this is known as eco-efficiency. This approach only makes sense when integrated into a holistic design process. Tools for value analysis and creativity should be used in parallel with environmental analysis tools (such as life cycle analysis) to effectively address the stated needs.
Eco-design relies on methods and tools that “extend” the traditional design process by incorporating some or all of the following elements:
Eco-design relies on methods and tools that “extend” the traditional design process by incorporating some or all of the following elements:
- Environmental assessment of products
- Implications of results in terms of recommendations or specifications
- Sustainability of the approach.
Eco-design, also known as eco-conception, is an approach that involves integrating environmental protection from the very beginning of designing goods or services. Its goal is to reduce the environmental impacts of products throughout their life cycle by considering various criteria. Here are some key aspects of eco-design:
- Raw material and energy consumption: Eco-design takes into account the quantities of virgin or recycled raw materials used in a product’s manufacturing, as well as the consumption of fossil or renewable energy throughout its life cycle.
- Emissions and pollution: It also evaluates emissions into water, air, and soil, as well as the toxicity associated with the product. Waste production is also considered.
- Alterations to natural environments: Eco-design considers the transformations that occur in natural habitats and living spaces due to the product’s life cycle.
Life Cycle Analysis
Life Cycle Analysis, also known as life cycle assessment, is a fundamental concept in eco-design. It requires considering significant environmental aspects of a product throughout all phases of its life cycle during the design process.
A product’s life cycle, from birth to end-of-life (“from cradle to grave”), can be broken down into four main stages:
- Extraction of raw materials: This stage involves obtaining the necessary raw materials for manufacturing the product.
- Production and distribution: During this phase, the product is manufactured, assembled, and distributed to consumers.
- Use phase: The product is utilized by consumers, and its environmental impact during this period is also considered.
- End-of-life: This stage involves disposal, recycling, or other forms of handling the product after its useful life.
A fairly typical view of the life cycle includes a transportation stage between the place of manufacture and the place of use. However, this perspective is too simplistic because, in reality, transportation steps should also be considered between different life phases (and sometimes even within phases).
Towards ecofabrication
In order for innovative production systems to be rationalized by combining traditional techno-economic approaches with sustainable ones, it will be necessary to include the environmental dimension in industry performance objectives. This will be particularly applied to the materials forming sector. For this purpose, two complementary visions are essential:
-
Firstly, these new approaches must be based on a mastery of the physical and mechanical behavior of materials throughout their entire life cycle, especially during the manufacturing (forming) phase. It is essential to understand the links between atomic structure and mechanical behavior during shaping. We focus on shaping using machines in a representative environment and associated modeling to predict material behavior in interaction with their environment and forming process.
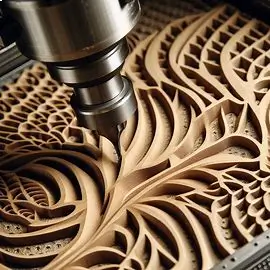
- Secondly, multidisciplinarity is indispensable for addressing “sustainable” questions, and the integration of multidisciplinary knowledge for decision-making in production systems constitutes the second key challenge. Therefore, methods and tools need to be proposed to integrate knowledge from economic sciences (decision support, risk management), human and social sciences (management, health, safety), as well as mastery of mathematical and computational tools (modeling, simulation, optimization) in the analysis of manufacturing system performance.
Access our online catalog
The SUNI platform, committed to environmental transition since 2017, positions itself to support you from eco-design to ecofabrication. Various processes have already been studied, including machining, metal additive manufacturing (material deposition, WAAM, BMD), polymer additive manufacturing (material extrusion, material projection), and composite shaping (thermo-stamping, 3D printing), as well as friction stir welding and flow drilling.
We can help you by providing training or supporting you with studies. Here is a non-exhaustive list of our services:
- Sustainability analysis of forming processes.
- Measurement of machine consumption and rejects.
- Life cycle inventory of manufacturing processes.
- Process LCA.
- Proposals for eco-manufacturing strategies.
PLATE-FORME TECHNOLOGIQUE SUNI
SUNI PFT
Campus de Ker Lann
Avenue Robert Schuman
35170 BRUZ , France
+33 02 99 05 93 18
contact@suni-pft.fr
Nous trouver
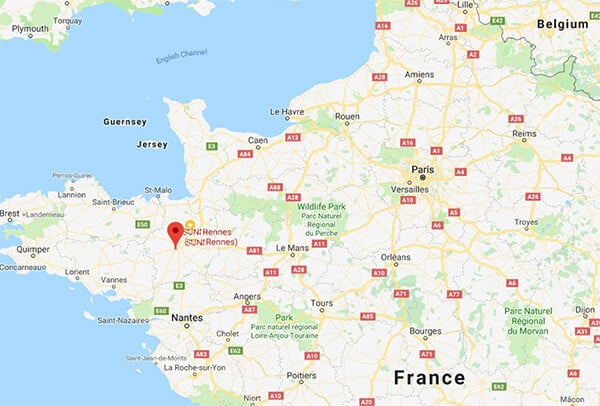
©Tous droits réservés SUNI 2018-2024
Design & Conception : Diginsol